Unmanned aerial systems (UAS) are a big topic these days, but not always in a good way. In October, Russia began an extensive campaign of attacks on the energy and electrical infrastructure across Ukraine using lethal drones and cruise missiles. Although many of Russia’s missiles and loitering munitions (including Shahed-136s and -131s supplied by Iran) are rudimentary, it has launched hundreds of them – sometimes in waves of up to a dozen or more at a time – with the aim of overwhelming Ukraine’s air defense systems. Ukraine claims to have shot down at least three-quarters of the drones and cruise missiles in these attacks, relying almost exclusively on its limited supply of RF- and IR-guided surface-to-air missiles. While a 75% kill rate is good for Ukraine, it’s the 25% of successful Russian attacks against its energy infrastructure that poses a serious problem for Ukraine. Russia is unlikely to abandon its drone attacks against Ukrainian power plants any time soon. In the long-term, defeating $20,000 drones with $200,000 surface-to-air missiles also puts Ukraine on the wrong side of the cost equation. But you fight with what you have – until you can get something that’s better.
Russia’s drone and cruise missile attacks on Ukrainian infrastructure have captured the world’s attention, but they not unique. In a future conflict, many countries could easily find themselves in a similar situation – facing an adversary’s weaponized drones. As the war in Ukraine is demonstrating, surface-to-air missiles and radar-directed AAA are not a complete answer to this problem. While they offer longer range and precision against aerial targets, they are primarily designed to attack fighter and bomber aircraft, as well as cruise missiles, rather than large number of relatively inexpensive drones. What is needed are systems that can rapidly fire multiple times (deep magazine) at low cost (non-kinetic) and preferably operate at the speed of light. In other words, what air defense units need to counter these drone and cruise missile attacks are directed energy weapons (DEWs).
Over the past decade, the US, Israel and several European nations have made significant advances in their development of DEWs – mainly high-energy laser (HEL) systems and high-power microwave (HPM) systems. In this article, we’re going to look at HPM technology, it’s history and current programs.
HPM weapons offer several advantages over other types of kinetic and non-kinetic weapons. HPM systems can typically fire in less than a second, and the latest generation of systems provide deep magazines (i.e., the ability to fire multiple times in a short period). HPM weapons can generate a range of effects on a target, from destroying or damaging sensitive electronic components to degrading their performance or forcing reboots and restarts. An HPM system delivers RF and microwave energy into a target’s processors and other logic components through the target’s RF and microwave antennas or into unshielded wiring and circuits that essentially act as apertures. Also, because an HPM system transmits from an antenna, it’s beam can be shaped to focus on a wide area or into a narrow beam. Depending on the type of aperture it uses wide antenna beam enables an HPM system to simultaneously attack several targets at one time and a narrow antenna beam can focus energy on a single target. Moreover, an HPM system equipped with an active electronically scanned array can change this beam shape very quickly as needed.
FROM SPARK GAP TRANSMITTERS TO PHASED ARRAYS
HPM weapons have a fascinating technological history, and it began when the US detonated a nuclear weapon 250 miles above the Pacific in 1962. As described by Stuart Moran in his 2012 article, “Historical Overview of Directed-Energy Work at Dahlgren,”¹ scientists noted, “The blast caused a large imbalance of electrons in the upper atmosphere that interacted with the Earth’s magnetic field to create oscillating electric fields over a large area of the Pacific. These fields were strong enough to damage electronics in Hawaii, a thousand miles away, and clearly demonstrated the effects of an electromagnetic pulse (EMP).”
This discovery raised eyebrows at the Pentagon, because if it could somehow be harnessed, the result would be a very effective non-kinetic tool in DOD’s arsenal. The question was how to realize such a weapon, which would require a means to generate field strengths of hundreds of thousands of volts (or more) per meter. Before exploring what came next, two facts are important to consider.
First, more than 60 years ago, DOD’s intention was to annihilate targets rather than just to disable them. And second, virtually all components in a target were analog rather than digital, which were (and still are) far more resistant to damage from high levels of EM energy than a tiny semiconductor device. Consequently, the need for massive amounts of RF energy was considered essential. Although this remains true today in some cases, massive amounts of energy aren’t always necessary or even desired when countering an adversary system using an HPM weapon.
As Air Power Australia think tank co-founder Dr. Carlo Kopp, who coined the term “E-bomb,” has noted in a 2012 article: “Expose any monolithic semiconductor device to voltages, whether transient or radio frequency, of more than the specification limits of several volts, and bad things usually happen. With a mains or battery power supply attached to the device, very little energy is needed to initiate a catastrophic electrical failure because the power supply is what delivers the killing blow.”²
Returning to the 1960s, Moran’s article explains how researchers at the Special Applications Branch at the Naval Weapons Laboratory at the Dahlgren (what is today the Naval Surface Warfare Center Dahlgren Division (NSWCDD) in Dahlgren, VA) got to work after the blast in the Pacific, and their efforts focused on high-power versions of spark-gap transmitters like those in the early days of radio broadcast. The RF generators were Hertzian oscillators, in which “a capacitor is charged to a high voltage, a switch is closed, and current flows in the circuit, causing the stored energy to oscillate between the electric field of the capacitor and the magnetic field of an inductor” until the breakdown voltage of the air between the spark gap is reached. At breakdown, a spark completes the LC circuit, oscillating at its resonant frequency. It can then pass the electromagnetic waves as electric oscillations.
The Hertzian-type devices were typically connected to a simple antenna and oscillated at low frequencies at 100 MHz or below. Measuring the output field of these devices was difficult because of ground effects, so a 100-meter ground plane of welded wire was laid out in a field with semi-underground trailers at each end to house high-voltage generators and diagnostics, which formed a basic measurement system. The sources often produced over a gigawatt of peak power and field strengths of several kilovolts per meter at the target sites.
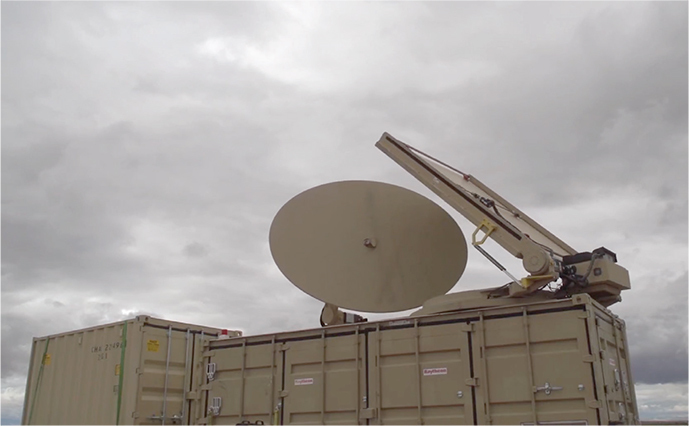
The Phaser is another counter-drone HPM system developed by Raytheon for AFRL. It is designed to take down drones either in single numbers or in swarms. AFRL photo
Moran describes many types of Hertzian devices that were designed, constructed and tested at Dahlgren throughout the 1970s. For example, the cavity oscillator “used a quarter-wavelength coaxial pipe that switched at one end to create an oscillating waveform.” Another type, the so-called “frozen-wave” generator, used “quarter-wave sections of cable that were charged to create a two-cycle waveform ‘frozen’ in the cable” and produced multi-kilowatt RF pulses in the megahertz frequency range with repetition rates of tens of kilohertz. Almost all the solutions created at Dahlgren using Hertzian oscillators could create at least 500 kV and produce large electric fields hundreds of meters away from the antenna.
Other types of devices included “vector inversion generators that used spiral-wound capacitive plates to generate the voltage” but didn’t require transformers, a significant improvement. A device called a Landecker ring mounted in the focal area of a parabolic dish antenna “used capacitors and inductors in a paddle-wheel arrangement charged in parallel and discharged in series.” The circular arrangement allowed the system to radiate as a dipole, effectively creating an antenna.
Or consider the Flux Compression Generator (FCG) that produces low-frequency wideband effects. Primed with an initial electrical starting current, “a high-velocity explosive is used to mechanically compress the magnetic field, transferring energy from the explosive into the magnetic field,” explains Kopp in an article about “E-Bombs.”² “While the FCG disintegrates during operation, it produces a powerful pulse of electrical current.” And when cascaded together, three FCGs can amplify power one hundredfold to deliver peak power outputs of many gigawatts.
Then there’s the Virtual Cathode Oscillator (Vircator) that produces brief pulses at tunable narrowband frequencies at very high-power levels. As Kopp’s “E-Bomb” article explains, the Vircator allows the power produced by the FCG to be precisely focused on a target area hundreds of meters away, although the most severe damage occurs within tens of meters.
In 1973, Dahlgren began the Special Effects Warhead (SEW) program to explore the feasibility of “‘burning out’ enemy radar and missile systems using single-shot, very high peak-power EMPs.” One of the goals was to determine if an electromagnetic warhead could be created that could disable electronics up to 1 mile away. The laboratory must have been both very interesting and very dangerous. As Moran’s article explains, “The high-voltage fields and sparking caused the workers to make a sign for one of the buildings: ‘Science Fiction Department’.’”
Little was known at the time about the vulnerability of foreign or US electronics and systems, and the transmitters used for testing could not produce fields high enough to test large targets, such as a Nike Hercules Radar System. To solve this problem, Moran explains, a trailer-based RF impulse system was constructed. It employed a “Marx generator-driven L-C oscillator charged charged at two million volts.” Known as the Transportable Oscillating Pulser System (TOPS), “it was connected to a large bounded-wave structure that produced uniform fields over a region large enough to place an entire radar or missile.” Moran goes on to write, “The electric field emitted from the system was so high that the air would arc unless a special bag of insulating gas (sulfur hexafluoride) was used until the radiating structure became large enough to transition to the normal atmosphere.”
MORE POWER PLEASE
At Dahlgren, Moran explains, it had become apparent that “the size, weight and cost of these systems were driven by the pulsed DC technologies needed to drive the system, not by the RF source itself. Consequently, more effort began to be devoted to the power-delivery technologies needed for many of the weapon concepts.” Pulsed-power components enabled energy to be stored over seconds – a long time in DE terms – and could be released in nanoseconds “to obtain a billion times increase in peak power.” Dahlgren officials convinced Navy leaders to initiate a Pulsed Power Technology Program in 1978 “to develop the power sources, energy storage systems, high-power switches and power conditioning systems needed for future weapons.”
Moran goes on to explain, “To provide large amounts of electrical prime power, new types of rotating machines were studied, including flywheels, conventional alternators, homopolar generators, rotary flux compressors and compensated pulsed alternators. These machines attempted to produce fast, high-power pulses using special materials to reduce losses, eddy currents and mechanical stresses.”
Over the remainder of the 1970s and into the 1980s, prime and RF power technologies continued to improve, as universities and several other DOD departments (including the Air Force Research Lab) joined Dahlgren in its HPM these efforts. However, the end of the Cold War weakened interest in directed energy weapons. The Pulsed Power Technology Program and the Navy’s Charged Particle Beam Program were canceled, although Dahlgren managed to cobble together funding to maintain a core technical competency that could be used later. Dahlgren is now one of two places in the US with a division specific to HPM technology development, the other being the Air Force Research Lab’s Directed Energy Directorate at Kirtland AFB, NM.
HPM RETURNS
By the mid 2000s, the world had long since begun its transition from analog to digital technology, and products once almost completely analog had become almost entirely digital. Very few of these electronic systems had been tested for their vulnerability to an HPM attack. The creation of massive clouds of EMP had seemingly become less important after the Soviet Union collapsed, so protecting electronics from damaging levels of RF energy also became less important.
This complacency did not last, however. As digital electronics became more widely used in US military systems and for controlling civilian infrastructure, such as electrical power plants, communications systems, and emergency and industrial systems, the potential that an adversary could attack these systems with HPM weapons became an increasing concern. Today, more than a dozen countries, most of them US allies, but also Russia and China, are developing HPM weapons technology – some of them with decades of experience. Consequently, defending against HPM attacks, has captured more attention from Pentagon leaders in recent years. The Joint Program Office for Special Technology Countermeasures (JPO/STC) at Dahlgren has performed intensive work on the vulnerability of digital systems to RF attack. As Moran’s article explains, the program also established a DoD-wide database of “vulnerability data, source designs and RF-effects information,” combining it with the information that with considerable foresight had been stored over the years. “In the late 1990s and early 2000s, Dahlgren initiated programs regarding the potential for RF attack using non-kinetic disruption” and also “developed RF payloads for UAVs and demonstrated their effectiveness in field tests.” It was DOD’s first demonstration of this type of HPM technology. To test them, two multistory buildings were reconfigured to reflect different types of building construction and electromagnetic shielding. Within them were “large complexes of electronics, computer networks, server systems, telephone systems, security systems, and various types of digital industrial controls [that]could be assembled, instrumented and exposed to attack.” This complex, called the Maginot Open Air Test Site (MOATS), was designed for testing the RF susceptibility of electronic equipment to potential HPM weapons and continues to be used to test target systems, as well as a variety of RF weapon technologies developed internally and by external and international organizations.
Traditionally, HPM systems concentrated on destroying vulnerable electronic components in a weapons system. But in the case of drones, drone swarms, or other targets, what’s required is only to either confuse the electronics or render them inoperable. Destroying them with high-power ultrawideband pulses is unnecessary, which means that an HPM system doesn’t necessarily need to transmit gigawatts of power to destroy a single target, as it would against other assets, but only what’s needed to render a sensor inoperable or affect the weapons systems navigation or flight controls. This capability has been demonstrated in one form or another for more than 15 years since Raytheon developed its Valiant Eagle system in 2006 and later BAE Systems Bofors HPM Blackout.
AFRL has been developing and evaluating at least four HPM demonstrator systems over the past few years. This includes Raytheon’s Phaser system, which transmits high levels of RF energy to a reflector antenna and can destroy multiple targets simultaneously. The conical shape of the beam means that a single pulse can attack several drones in midair at once, making Phaser useful against drone swarms.
AFRL’s Tactical High Power Operational Responder (THOR) HPM system, developed by Leidos, BAE Systems and Verus Research, has demonstrated the ability to disable more than 100 drones at a time. AFRL is also progressing on a more advanced version of THOR to disable drone swarms that threaten military bases. The next-generation platform is named Mjölnir as an homage to the mythical god Thor’s hammer. AFRL has awarded Leidos a $26 million contract to develop the Mjölnir prototype and deliver it in early 2024.
Finally, AFRL is working with Raytheon to develop the Counter-Electronic High Power Microwave Extended Range Air Base Defense (CHIMERA) system. Unlike the Phaser and THOR systems, which operate against targets a close range, CHIMERA (as its name suggests) is designed to attack airborne targets at longer distances.
It’s important to note that many of these systems, including THOR and Phaser, use vacuum tube technology to generate the required radiated power. Most of them are also relatively large and best suited to fixed site applications, such as defending military bases against drones and rocket attacks.
AFRL and NRL have been developing another class of HPM weapons that is smaller and designed for use on missiles and UASs. The Counter-electronics High Power Microwave Advanced Missile Project (CHAMP), demonstrated in 2012 by Boeing and AFRL, uses the body of an AGM-86 Conventional Air Launched Cruise Missile (CALCM) to carry a Raytheon-developed HPM payload that can deliver multiple HPM “shots” per sortie. The High-Powered Joint Electromagnetic Non-Kinetic Strike Weapon, known as HiJENKS, also uses microwave technology to disable an adversary’s electronic systems. HiJENKS is the successor to CHAMP and uses smaller and more rugged HPM technology that can be integrated into a wider range of carrier systems. Funded jointly by the Air Force Research Laboratory and the Office of Naval Research, the five-year HiJENKS program completed “capstone” tests at Naval Air Station China Lake (China Lake, CA) last summer.
POWERED BY GAN
The most recent development in HPM technology is the use of active phased arrays and solid-state (gallium nitride – GaN)-based RF power amplifiers rather than vacuum tubes to generate the required levels of RF power. One system, called Leonidas, was developed independently by a relatively new company named Epirus. Started in 2018, with facilities in Los Angeles and McLean, VA, Epirus is leveraging a select set of core technologies for both defense and commercial applications.
Leonidas is the company’s scalable HPM offering. Initially developed in a trailer configuration, the company has also tested an airborne pod for UASs, and in October it disclosed a mobile version integrated onto a Stryker vehicle. Like an AESA system, Leonidas uses beam steering to focus its energy on a target or targets while defining no-fly zones to allow friendly forces to continue operating. Leonidas can be mounted on a comparatively small ground-based vehicle and can fire thousands of “rounds” per second. Leonidas has open systems architecture and relies on GaN-based line-replaceable amplifier modules and rapidly fires a barrage of unique waveforms to exploit the frequencies that make a UAS target susceptible.
The company stresses that rather than confusing the drone’s digital electronics by interrupting their communication of satellite navigation signals, Leonidas is better described as an EMP generator that envelopes the target with electromagnetic energy that covers very low to very high frequencies, “DC to daylight” as EMP is often described. “We don’t care what frequency a drone operates on”, says Andrew Lowery, chief product officer at Epirus. “We are an EMP system, so frequencies do not matter. Trying to disable the data links or GPS is comparatively simple, and lots of companies can do that. Instead, we blanket the target from a long distance, way beyond kinetic ranges or even THOR.”
Although the company defines the range of Leonidas only as a “tactically-relevant” distance, this typically means something like 10 km. “Our beam is actually not very narrow; its 3-dB beamwidth is about 6 deg.,” he continues. “If I want to take out a fleet of drones, we sweep the sky at a thousand times per second so any drone moving into that will encounter a wall of energy as we paint the beam across the sky.
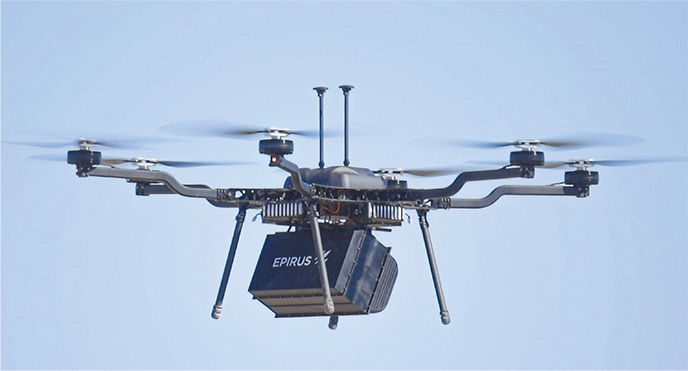
Epirus has developed a family of HPM systems based on its original Leonidas counter-drone system (below, left). It teamed with General Dynamics to introduce a Stryker-mounted system (top, left), and it has introduced a podded system for drones (above). Epirus
“We just want to put a gigantic e-field there, and as cavities and other entry points are prone to vulnerability at some frequencies more than others, we create shields of energy rather than shooting a bullet of energy. Anything that flies into the shield gets disrupted. Even with little gaps and various other entry points, if you put 10 or 20 volts across a switch, it will mess up the electronics enough to shut it down. That’s the power of EMP. It can affect a computer, a camera, engine control, or any other circuit.”
Leonidas uses the company’s proprietary SmartPower power management technology that optimizes RF performance for generating high-power pulses while ensuring the system does not overheat. The SmartPower technology platform, a proprietary combination of hardware, software and machine-intelligent algorithms, uses real-time artificial intelligence and machine learning, and can reduce power consumption by up to 70%, which lowers system power demands, the need for very high-power sources, and requires needs less cooling than vacuum-tube-based systems.
Digital technology oversees the conversion of energy, from AC to DC, for example. “Power is enhanced through a digital architecture, because all of those non-linear circuits in the target are surrounded by a passive network that places it in the right bias condition,” says Lowery. “It optimizes the performance of the non-linear circuit, and we have a range of dynamic control, so we go beyond just what a passive static system can do. That is, we use machine learning to manage the circuit by modulating drain, gate and input signals to optimize every conversion metric.
“We also perform envelope tracking, predistortion and algorithms on the gate for pulsed applications, rise and fall times, etc.,” Lowery explains. “This eliminates a vast amount of waste that traditionally occurs with nonlinear conversion, and it increases efficiency and reduces heat, so the cooling required is much less. We do everything we can to maximize EIRP on the target, as each of the channels doesn’t have the EIRP required by a single-channel approach, such as THOR.”
Because the system is software-defined, waveforms can be modified in bandwidth and other characteristics in real time to address a specific target in a crowded electromagnetic environment. This means Leonidas can deal with an enemy drone near a friendly asset while simultaneously mitigating large swarms of drones. Artificial Intelligence and Machine Learning also enable the Leonidas system to learn over time, as it is exposed to new target data. Software updates can be delivered over the air to optimize characteristics to achieve positive effects against new targets.
REAL-WORLD PROBLEMS
HPM technology is maturing rapidly and, just as it did in the 1970s and 1980s, the demand signal from the operational community is increasing – this time for air defense applications and for airborne weapons that can attack the many non-kinetic soft spots (i.e., unshielded wiring and unprotected digital components) in an adversary’s sensor-to-shooter networks.
The Ukrainian Armed Forces could certainly benefit from many of these HPM systems right now, but that seems unlikely even though the Russian drones and cruise missiles would be an excellent field test for them. THOR and PHASER reportedly have been deployed outside the US as part of their evaluations, while Leonidas has not. Lowery at Epirus says the company has built four ruggedized prototypes of Leonidas that “could” become LRIP-ready and could be deployable and sent to Ukraine in 2023. As the drone and missile strikes against Ukraine are expected to increase over the next few months, however, Ukraine’s need for HPM weapons to counter them will certainly increase, as well. They could form one part of a multi-layered air defense solution to an complex problem.